Refractive index profile tailoring of multimode optical fibers for the spatial and spectral shaping of parametric sidebands
To generate a parabolic index profile, optical fibers are drawn from performs, which are often obtained by chemical vapor deposition of dopants. A central index dip may appear in the deposition process, owing to the volatilization of silica, and dopants in the innermost layers. This effect is usually unwanted, and it can be circumvented by increasing the concentration of the more volatile dopant (see Fig. 1)
In this work, we explore the possibility to control spatio-temporal beam shaping in multimode fibers (MMFs) by a suitable tailoring of the refractive index profile of the fiber. We unveil the central role played by the refractive index profile for the control of spatial and spectral properties of nonlinear wave propagation in MMFs. In particular, we reveal that a relatively small localized depression (or dip) in the refractive index of a GRIN fiber has a significant impact both on the Kerr beam self-cleaning, and most strikingly, on the parametric sideband generation.
It is well known that any deviation of the refractive index profile of a GRIN MMF from the ideal parabolic shape has direct consequences on the spacing among propagation constants of its modes. Here we show that this simple consideration has dramatic consequences on the mode selection properties of parametric sidebands, as a result of the disruption of the collective self-imaging effect, which is a typical characteristic of multimode wave propagation in ideal GRIN fibers.
Specifically, we show that a central index dip in the refractive index profile of GRIN fibers may be exploited to inspire new ways of shaping the spatial and spectral domains of light waves generated by four-wave mixing. In GRIN fibers with an ideal parabolic index profile, geometric parametric instability (GPI) is pumped by a bell-shaped self-cleaned beam, which translates its spatial profile into all sidebands [1]. These sidebands are generated by quasi-phase matching (QPM) via the dynamic grating resulting from the Kerr effect and pump self-imaging. The presence of a refractive index dip breaks the oscillation synchronism among fundamental and higher-order modes, which spoils the QPM between the pump and sidebands. As a result, to obtain phase matching, the sidebands and the pump need to be carried by different modes of the fiber. Hence, a particular inter-mode four-wave-mixing (IMFWM) process prevails as the sideband generation mechanism. Quite remarkably, the central positions of these IMFWM sidebands, experimentally measured with the fiber having a central defect, still nearly coincide with sideband positions obtained with the perfect GRIN fiber, as derived from a GPI analysis. On the other hand, the index perturbation leads to a broadening of sideband spectral bandwidths (see Fig.2).
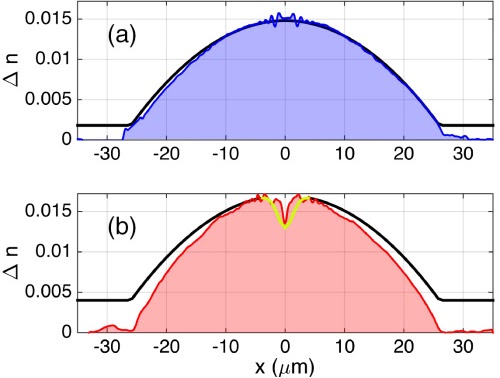
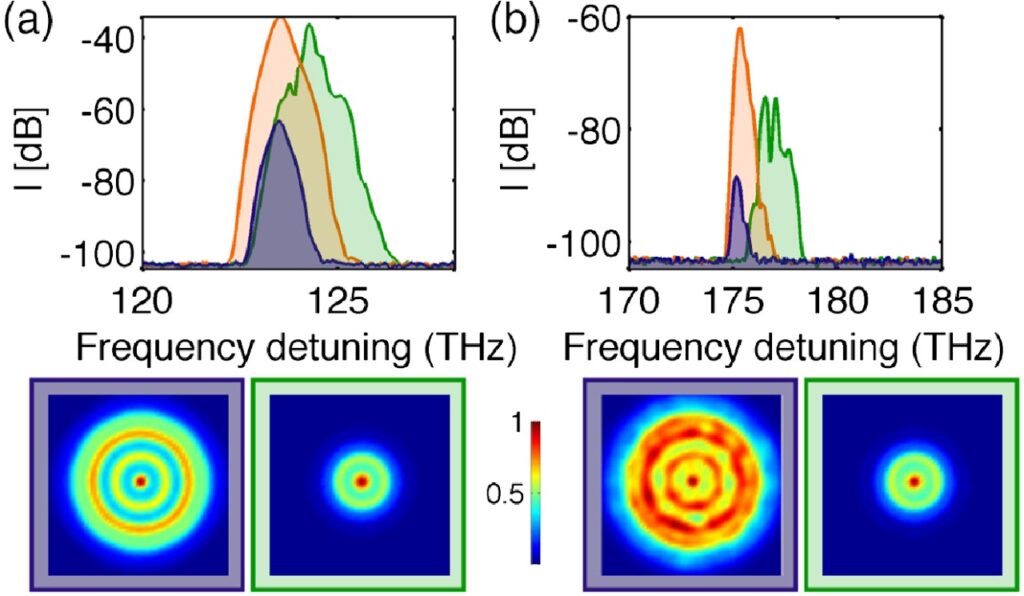
We used two different standard 10-m-long GRIN MMFs: their refractive index profiles, illustrated in Fig. 1, were close to the ideal parabolic shape for the first fiber, and exhibited a dip at the center of the core for the second fiber. We pumped the fibers by an amplified Nd:YAG microchip laser, emitting pulses at 1064 nm with a temporal duration of 900 ps and a repetition rate of 30 kHz. We focused a linearly polarized Gaussian beam at the input face of the fibers with a full-width at half-maximum intensity (FWHMI) diameter of 30 ΞΌm. Such coupling method allowed us in principle to excite a large number of modes, thanks also to the unavoidable presence of random linear mode coupling among all modes.
First we compared Kerr beam self-cleaning in a GRIN MMF with a central dip, with the case of a GRIN MMF without a dip. Figure 3 illustrates the variation, versus input guided peak power ππβπ, of the output beam diameter from the fiber with a dip, measured at the FWHMI. As can be seen, for ππβπ above 20 kW, the output beam diameter is nearly halved with respect to its value at low powers. The insets of Fig. 3 illustrate the corresponding evolution of the spatial shape of the beam for selected input power values. In spite of the presence of the dip in the refractive index profile of the GRIN fiber, Kerr-induced beam self-cleaning toward a bell-shaped spatial pattern could still be clearly observed. although the threshold power was six times larger than in the case of an ideal parabolic index profile.

Next, we considered the generation of parametric sidebands: in Fig. 4 we compare experimental optical spectra obtained in the two multimode GRIN fibers with (blue curve) and without (red curve) the dip, for the same input guided peak power of ππβπ=36 kW. As can be seen, our experiments clearly demonstrate that only the pump beam at 1064 nm exhibits a spatial distribution with a bell-shaped profile, as a result of the Kerr self-cleaning.
A series of transverse beam profiles corresponding to different spectral components, which were selected by using various 10-nm-wide bandpass filters with different center wavelengths, are displayed in Fig. 5 for the case of the GRIN fiber with a dip. The generated sidebands are carried by high-order modes: the larger the order of the peak, the higher the order of the associated spatial mode.
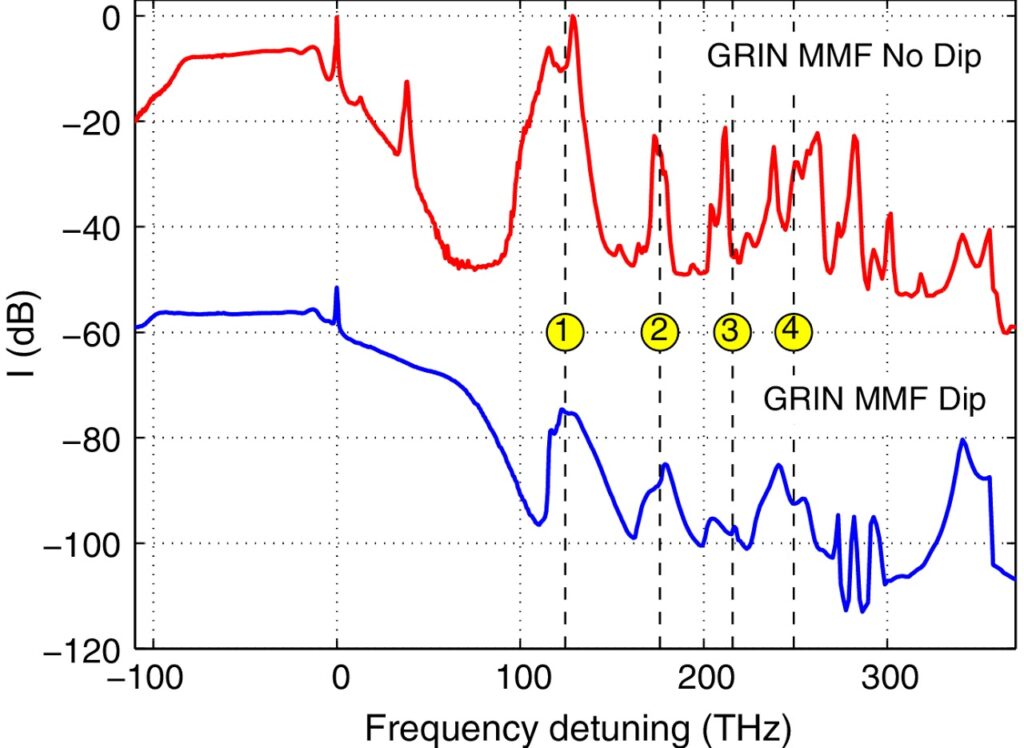

We also observed supercontinuum, generated by the interplay of stimulated Raman scattering and multimode solitons appearing on the Stokes side of the pump. However, the spatial profile at Stokes frequencies was no longer carried by the fundamental mode, in contrast with the case of a nearly ideal GRIN MMF [2]. Figure 6 summarizes the supercontinuum spectrum and the associated transverse beam shapes at some selected wavelengths from the GRIN fiber with dip. In this different series of experiments, it is visible how the pump beam only has a bell-shaped beam, whereas the supercontinuum is carried mainly by a beam shape with two lobes. This result is of particular interest, and it shows how a defect in a graded index fiber can lead to the generation of a supercontinuum with a beam shape with two main lobes.
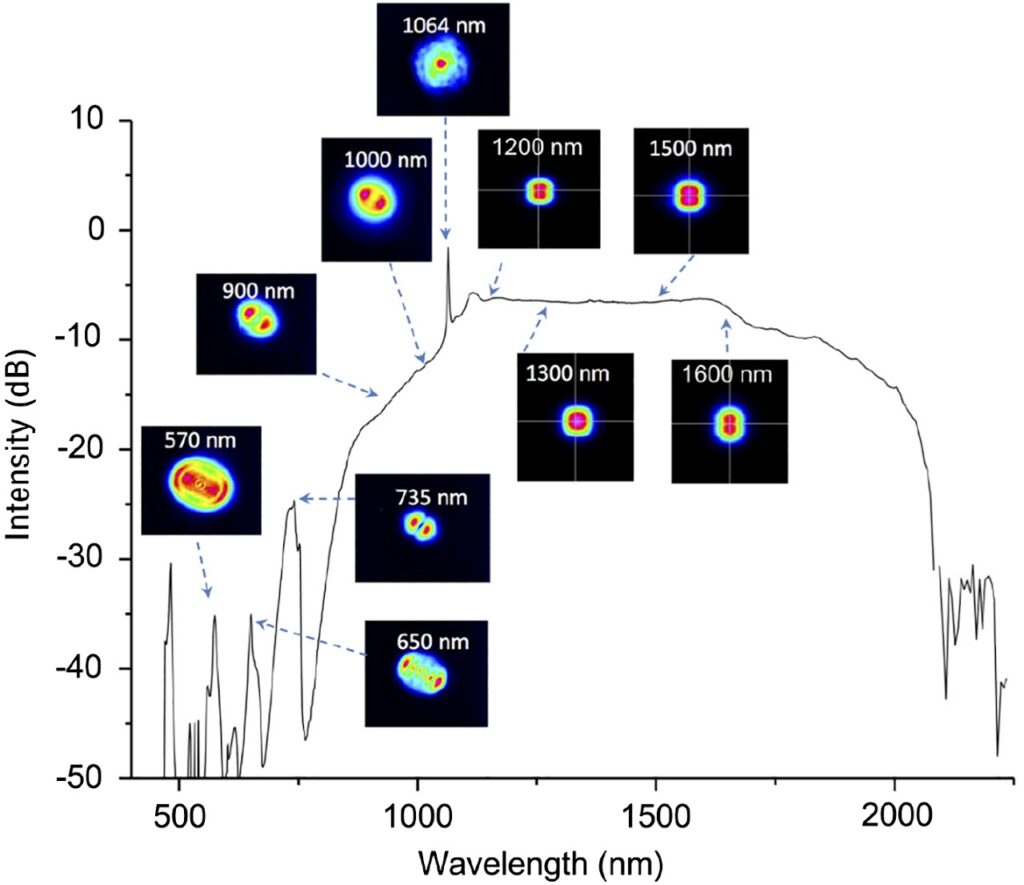
- K. Krupa, A. Tonello, A. BarthΓ©lΓ©my, V. Couderc, B. M. Shalaby, A. Bendahmane, G. Millot, and S. Wabnitz, βObservation of geometric parametric instability induced by the periodic spatial self-imaging of multimode waves,β Phys. Rev. Lett. 116, 183901 (2016).
- K. Krupa, C. Louot, V. Couderc, M. Fabert, R. Guenard, B. M. Shalaby, A. Tonello, D. Pagnoux, P. Leproux, A. Bendahmane, R. Dupiol, G. Millot, and S. Wabnitz, βSpatiotemporal characterization of supercontinuum extending from the visible to the mid-infrared in a multimode graded-index optical fiber,β Opt. Lett. 41, 5785β5788 (2016).